Friday, August 17, 2007
Reflections
For this past grading period, I realized that high grades aren't that easy to achieve when the subject's a combination of science and math.
My performances this grading period aren't good because I couldn't cope up with the lessons. It is because my foundation in mathematics in my elementary days are not good enough. It can not tranform formula, especially when fractions and square roots are involved.
I did my best in every paper work that Mr. Mendoza asked us to do like the paper that asked us to make a research work about the nature of light. And every output was made very satisfactory. It may not please Mr. Mendoza that much but surely efforts were used. The tests were rather hard for I did not study. Listening in the discussions weren't enough.
As I conclude the posts in my physics journal, in every thing rating that I get from what I did is acceptable and I will not complain all about it.
I wanted to learn at 7:45 PM.
Thursday, August 9, 2007
Eye and Camera: A Comparison; from:http://health.indiamart.com/eye-care/anatomy-of-eye.html
Anatomy and Functioning of the Eye
Parts of the Eye
Cornea -- the transparent front window of the eye. The cornea transmits and focuses light into the eye.
Iris -- the colored part of the eye. The iris helps regulate the amount of light that enters the eye.
Pupil -- the dark center in the middle of the iris. The pupil changes size in response to various degrees of illumination to control the amount of light that is let into the eye.
Lens -- the transparent structure behind the iris that focuses light rays onto the retina.
Retina -- the nerve layer that lines the back of the eye. The retina senses light and creates impulses that are sent through the optic nerve to the brain.
Macula -- a small specialized area in the retina that contains special light sensitive cells. The macula allows us to see fine details clearly.
Optic Nerve -- the nerve that connects the eye to the brain. It carries the impulses formed by the retina to the brain, where it is interpreted as images.
Vitreous -- the clear, jelly-like substance that fills the middle of the eye.
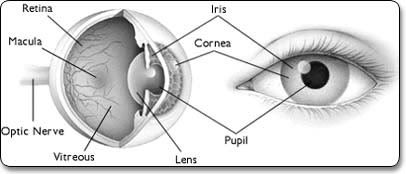
Eye as a Camera
The function of our eyes is to enable us to see clearly the objects in our surroundings at variable distances and under various conditions of lights. This function is achieved by a very complex arrangement of structures in the eye. Our eye can be thought of as a very advanced camera. There are many similarities between our eye and a camera. Like in a camera the aim is to provide a well-focused image of the object onto the film at the back of the camera. Similarly in the eye, the retina, which is situated towards the back of the eye, works as the photographic film. The image is formed there and then the signal is sent from there to our brain through the optic nerve, and thus we perceive the objects around us. In fact, the retina is much more advanced than a photographic film because it can automatically change its sensitivity depending upon the amount of illumination present.
The rays of light enters the eye and passes through cornea and lens, which converge them so that it gets focused at the retina and a sharp image is formed. This function is akin to that of the lens of a camera. Once again the eye is superior, because the lens of the eye has the property to automatically adjust its power depending upon the location of the object of interest. Therefore, whenever we see from distance to near object, the lens of eye increases its curvature and thus is able to focus the image clearly onto the retina. The aperture (pupil) in the colored part (iris) of the eye is also adjustable according to the illumination of the surroundings. This is akin to the aperture of the diaphragm in a camera.
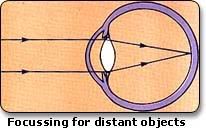
How does the rays of light get focused in a normal eye?
In a normal eye, the rays of light coming from a distant object gets focused by the cornea and the lens of the eye onto the retina and form a sharp image. When looking at a near object, the lens of the eye becomes more convex (accommodation) and thus its converging power is increased. This helps in focusing the near object clearly on the retina.
I wanted to learn at 9:59 PM.
Wednesday, August 8, 2007
Fiber Optics; from: http://electronics.howstuffworks.com/fiber-optic.htm
Fiber-optic lines are strands of optically pure glass as thin as a human hair that carry digital information over long distances. They are also used in medical imaging and mechanical engineering inspection.

What exactly are fiber optics?
Fiber optics (optical fibers) are long, thin strands of very pure glass about the diameter of a human hair. They are arranged in bundles called optical cables and used to transmit light signals over long distances.

Parts of a single optical fiber:
Core - Thin glass center of the fiber where the light travels
Cladding - Outer optical material surrounding the core that reflects the light back into the core
Buffer coating - Plastic coating that protects the fiber from damage and moisture
Hundreds or thousands of these optical fibers are arranged in bundles in optical cables. The bundles are protected by the cable's outer covering, called a jacket.
Optical fibers come in two types:
Single-mode fibers
Multi-mode fibers
How Does an Optical Fiber Transmit Light?
Suppose you want to shine a flashlight beam down a long, straight hallway. Just point the beam straight down the hallway -- light travels in straight lines, so it is no problem. What if the hallway has a bend in it? You could place a mirror at the bend to reflect the light beam around the corner.
What if the hallway is very winding with multiple bends? You might line the walls with mirrors and angle the beam so that it bounces from side-to-side all along the hallway. This is exactly what happens in an optical fiber.

The light in a fiber-optic cable travels through the core (hallway) by constantly bouncing from the cladding (mirror-lined walls), a principle called total internal reflection. Because the cladding does not absorb any light from the core, the light wave can travel great distances. However, some of the light signal degrades within the fiber, mostly due to impurities in the glass. The extent that the signal degrades depends on the purity of the glass and the wavelength of the transmitted light (for example, 850 nm = 60 to 75 percent/km; 1,300 nm = 50 to 60 percent/km; 1,550 nm is greater than 50 percent/km). Some premium optical fibers show much less signal degradation -- less than 10 percent/km at 1,550 nm.
A Fiber-Optic Relay System
To understand how optical fibers are used in communications systems, let's look at an example from a World War II movie or documentary where two naval ships in a fleet need to communicate with each other while maintaining radio silence or on stormy seas. One ship pulls up alongside the other. The captain of one ship sends a message to a sailor on deck. The sailor translates the message into Morse code (dots and dashes) and uses a signal light (floodlight with a venetian blind type shutter on it) to send the message to the other ship. A sailor on the deck of the other ship sees the Morse code message, decodes it into English and sends the message up to the
captain.
Fiber-optic relay systems consist of the following:
Transmitter - Produces and encodes the light signals
Optical fiber - Conducts the light signals over a distance
Optical regenerator - May be necessary to boost the light signal (for long distances)
Optical receiver - Receives and decodes the light signals
Transmitter
The transmitter is like the sailor on the deck of the sending ship. It receives and directs the optical device to turn the light "on" and "off" in the correct sequence, thereby generating a light signal.
The transmitter is physically close to the optical fiber and may even have a lens to focus the light into the fiber. Lasers have more power than LEDs, but vary more with changes in temperature and are more expensive. The most common wavelengths of light signals are 850 nm, 1,300 nm, and 1,550 nm (infrared, non-visible portions of the spectrum).
Optical Regenerator
As mentioned above, some signal loss occurs when the light is transmitted through the fiber, especially over long distances (more than a half mile, or about 1 km) such as with undersea cables. Therefore, one or more optical regenerators is spliced along the cable to boost the degraded light signals.
An optical regenerator consists of optical fibers with a special coating (doping). The doped portion is "pumped" with a laser. When the degraded signal comes into the doped coating, the energy from the laser allows the doped molecules to become lasers themselves. The doped molecules then emit a new, stronger light signal with the same characteristics as the incoming weak light signal. Basically, the regenerator is a laser amplifier for the incoming signal.
Optical Receiver
The optical receiver is like the sailor on the deck of the receiving ship. It takes the incoming digital light signals, decodes them and sends the electrical signal to the other user's computer, TV or telephone (receiving ship's captain). The receiver uses a photocell or photodiode to detect the light.
Advantages of Fiber Optics
Why are fiber-optic systems revolutionizing telecommunications? Compared to conventional metal wire (copper wire), optical fibers are:
Less expensive - Several miles of optical cable can be made cheaper than equivalent lengths of copper wire. This saves your provider (cable TV, Internet) and you money.
Thinner - Optical fibers can be drawn to smaller diameters than copper wire.
Higher carrying capacity - Because optical fibers are thinner than copper wires, more fibers can be bundled into a given-diameter cable than copper wires. This allows more phone lines to go over the same cable or more channels to come through the cable into your cable TV box.
Less signal degradation - The loss of signal in optical fiber is less than in copper wire.
Light signals - Unlike electrical signals in copper wires, light signals from one fiber do not interfere with those of other fibers in the same cable. This means clearer phone conversations or TV reception.
Low power - Because signals in optical fibers degrade less, lower-power transmitters can be used instead of the high-voltage electrical transmitters needed for copper wires. Again, this saves your provider and you money.
Digital signals - Optical fibers are ideally suited for carrying digital information, which is especially useful in computer networks.
Non-flammable - Because no electricity is passed through optical fibers, there is no fire hazard.
Lightweight - An optical cable weighs less than a comparable copper wire cable. Fiber-optic cables take up less space in the ground.
Flexible - Because fiber optics are so flexible and can transmit and receive light, they are used in many flexible digital cameras for the following purposes:
Medical imaging - in bronchoscopes, endoscopes, laparoscopes
Mechanical imaging - inspecting mechanical welds in pipes and engines (in airplanes, rockets, space shuttles, cars)
Plumbing - to inspect sewer lines
Physics of Total Internal Reflection
When light passes from a medium with one index of refraction (m1) to another medium with a lower index of refraction (m2), it bends or refracts away from an imaginary line perpendicular to the surface (normal line). As the angle of the beam through m1 becomes greater with respect to the normal line, the refracted light through m2 bends further away from the line. At one particular angle (critical angle), the refracted light will not go into m2, but instead will travel along the surface between the two media (sine [critical angle] = n2/n1 where n1 and n2 are the indices of refraction [n1 is greater than n2]). If the beam through m1 is greater than the
critical angle, then the refracted beam will be reflected entirely back into m1 (total internal reflection), even though m2 may be transparent!
In physics, the critical angle is described with respect to the normal line. In fiber optics, the critical angle is described with respect to the parallel axis running down the middle of the fiber. Therefore, the fiber-optic critical angle = (90 degrees - physics critical angle). In an optical fiber, the light travels through the core (m1, high index of refraction) by constantly reflecting from the cladding (m2, lower index of refraction) because the angle of the light is always greater than the critical angle. Light reflects from the cladding no matter what angle the fiber itself gets bent at, even if it's a full circle!
Because the cladding does not absorb any light from the core, the light wave can travel great distances. However, some of the light signal degrades within the fiber, mostly due to impurities in the glass. The extent that the signal degrades depends upon the purity of the glass and the wavelength of the transmitted light (for example, 850 nm = 60 to 75 percent/km; 1,300 nm = 50 to 60 percent/km; 1,550 nm is greater than 50 percent/km). Some premium optical fibers show much less signal degradation -- less than 10 percent/km at 1,550 nm.
I wanted to learn at 2:30 PM.
Friday, August 3, 2007
Mirror
Ray Diagram for Concave Mirrors
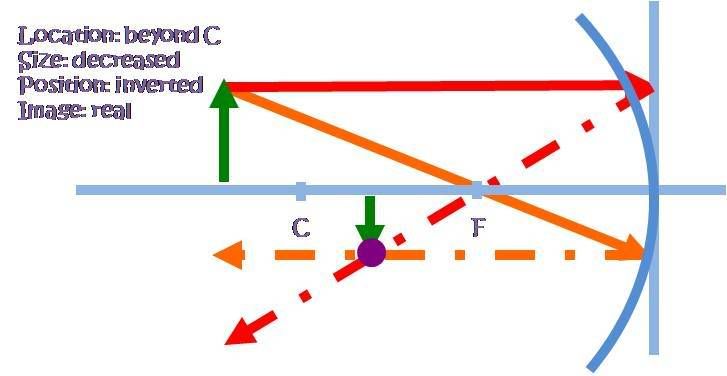
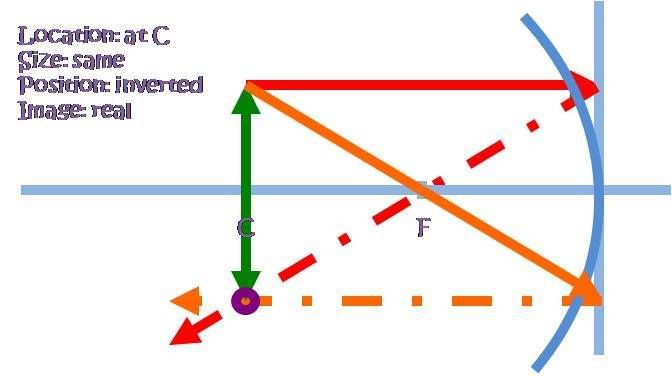
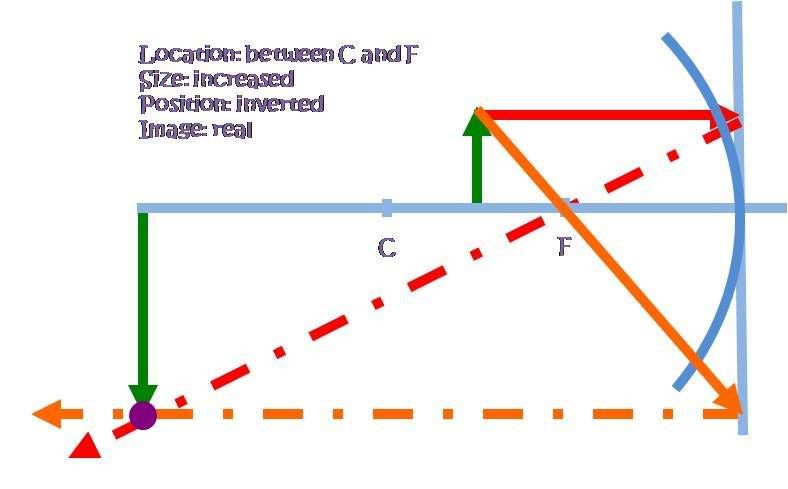

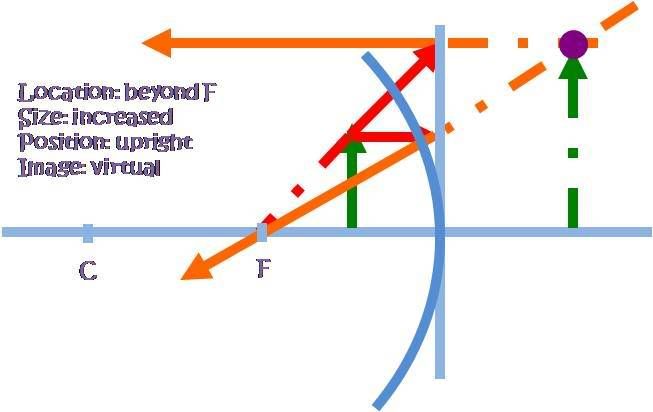
Ray Diagaram for Convex Mirrors

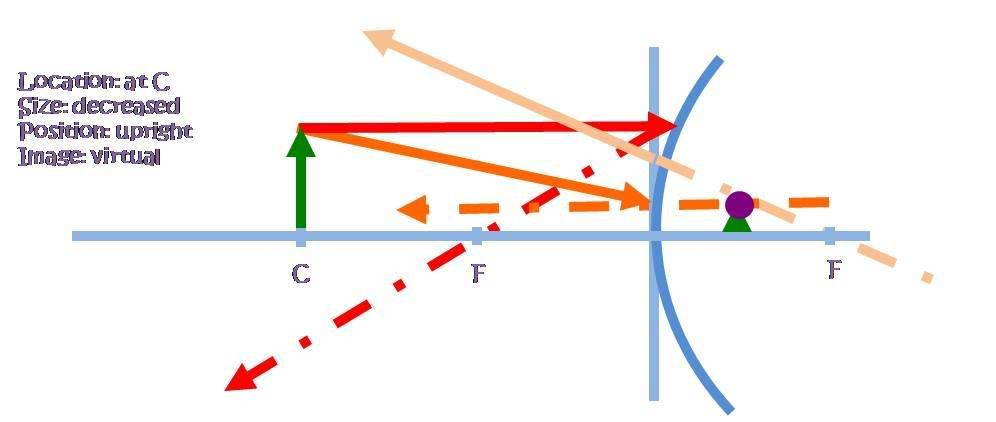
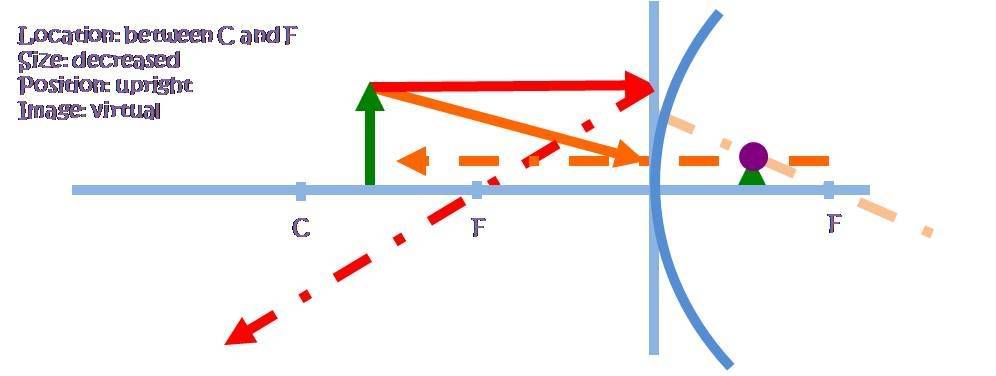
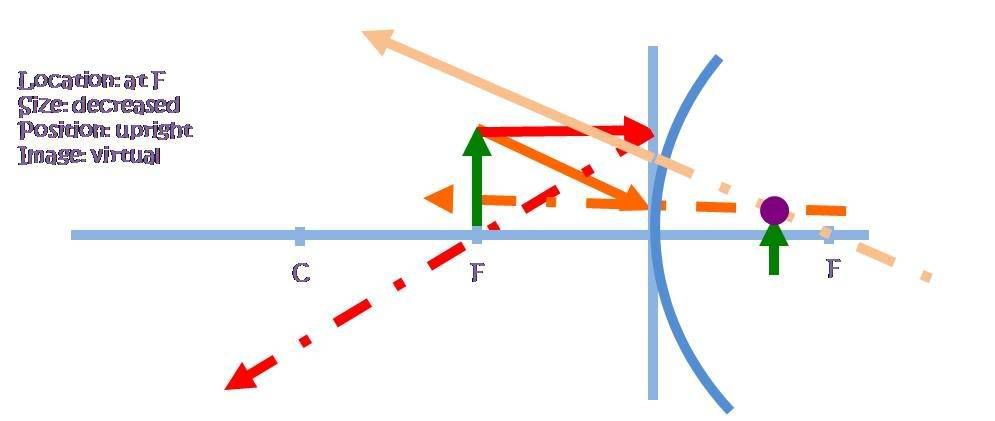

I wanted to learn at 10:37 PM.